Preparation and Spectroscopic Characterization of the Classical Coordination Compounds Copper(II) and Silver(II) Picolinate. A Comparison of a First and Second Transition-Series Elements in the Same Family.
Preparation and Spectroscopic Characterization of the Classical Coordination Compounds Copper(II) and Silver(II) Picolinate. A Comparison of a First and Second Transition-Series Elements in the Same Family.
By: Sara Adelman and Agust Olafsson
Introduction:
Coordination compounds are of immense importance in the biological and synthetic world and have a wide range of uses: from naturally occurring enzymes to synthetic drugs to indispensable industrial products1. The variety of uses of the coordination compounds is derived from their structural diversity. Classical coordination compounds have a transition metal center that acts as an electron acceptor to the electron-donating ligands, which together are called the coordination sphere2. These compounds can be synthesized by directly adding ligands to a metal, or more commonly through a ligand substitution reaction. In a ligand substitution reaction the metal center exchanges preexisting ligands for new ligands without changing the oxidation state of the central atom3. Ligands act as lone pair electron donors and are therefore Lewis bases; where the ligand-donated electrons occupy empty metallic orbitals, forming a coordinate covalent bond. Coordination compounds containing the group 11 elements copper, silver, or gold, collectively known as the coinage metals, have great potential for practical applications, including light emitting diodes, lubricant additives, catalysis, ionic liquids, and liquid crystals4. In this study, two picolinate coordination compounds are characterized and compared using either copper or silver ion in the 2+ oxidation as the central complex atoms.
To understand the electronic and structural characteristics of any coordination compound, some basic molecular details must first be determined. The coordination number and geometry of the compound, two crucial identifiers, are influenced by three main factors: the size of the central metal atom, steric interactions between ligands, and the electronic interactions between ligands and the metal center5. Larger metal atoms can accommodate more substituents and therefore can have higher coordination numbers.
Ligands may impose geometry onto the entire molecule if the ligand it is a very strong field ligand and therefore high in the spectrochemical series. Another way ligands shape the molecule is the number of lone pair electrons the ligand can readily donate. When ligands are able to donate more than one lone pair of electrons, the coordination compound formed between the ligand and metal is an especially stable compound, a chelate. Typically, the electron-donating atom in a chelating agent is sulfur, oxygen, or nitrogen6.
The ligand used in both of the coordination compounds in this experiment was picolinate, the deprotanated anion of picolinic acid. Picolinate is high in the spectrochemical series, a good chelator and has a rigorously square planar geometry.
Silver(II) and copper(II) picolinate are two chelates that are readily isolated in a laboratory setting. However, the silver(II) cation must be generated in situ from silver(I). Silver(II) is a powerful oxidizing agent that will oxidize water to produce gaseous oxygen in solution if a strong Lewis base is not present to form a complex. The potency of silver(II) as an oxidizer is derived from the its increased ligand splitting energy, which is greater than that of copper(II) as predicted by the periodic trend of increasing ligand splitting energy down a transition series5.
Four instrumental techniques were used to obtain a thorough analysis of both synthesized compounds. Infrared (IR) spectroscopy was used to confirm the successful synthesis of the picolinate compounds and characterize their molecular structures, electronic absorption spectroscopy to define their electronic structures, electron paramagnetic resonance (ESR) to observe electron densities, and density function theorem (DFT) calculations to describe the products’ geometries.
Experimental:
Silver(II) Picolinate– Four solutions were prepared: AgNO3 (0.2023 g, 0.001191mol) in Millipore purified water (20 mL); (NH4)S2O8 (0.1541 g, 6.75×10-3 mol) in Millipore purified water (20 mL); Na2CO3 (0.2552 g, 0.002408 mol) in Millipore purified water (20 mL); and Picolinic acid (0.2965 g, 0.002408 mol) in the minimal amount of Millipore purified water. The AgNO3 solution was added to the picolinic acid solution, followed by the (NH4)2S2O8 solution and then the Na2CO3 solution. The resulting suspension solution was stirred for 5 minutes at room temperature and then collected by vacuum filtration and washed with Millipore purified water. The solid was vacuum dried for 24 hours and collected as a red-orange powder (68%).
Copper(II) Picolinate– Two solutions were prepared: Cu2(CH3CO2)4(H2O)2 (0.211 g, 5.04×10-4 mol) in Millipore water (10mL) and picolinic acid (0.2482 g, 2.02×10-3 mol) in Millipore water (10 mL). The Cu2(CH3CO2)4(H2O)2 solution was then added to the picolinic acid solution. A blue suspension liquid formed and was stirred for 20 minutes with light heating. The suspension product was collected by vacuum filtration and washed with Millipore water. The solid was vacuum dried for 24 hours and collected as a blue powder (91%).
Solid state and solution state electronic absorbance spectra were measured by a Cary Bio300 UV/Vis spectrophotometer with a range of 300 to 900 nm, in 0.1 nm intervals at a rate of 200 nm/min. Base line corrections for the solid state were recorded as mineral oil on filter paper, and Millipore water for the solution spectra. IR spectra were recorded on a Midac-M series FTIR spectrometer between 4000 and 500 cm-1. Samples were prepared in a mineral oil mull between two NaCl plates. Electronic spin resonance spectra were recorded by a JOEL JES-TE100 ESR in the solid state samples in a quartz tube at room temperature. The density functional calculations were made semi-empirically using Spartan10.
Results:
Copper(II) picolinate (Figure 1a) was synthesized forming a blue powder solid with a 91% yield. Silver(II) picolinate (Figure 1b) was isolated as a blue crystalline solid with a percent yield of 67% following its synthesis. To obtain silver(II) picolinate, silver(I) was oxidized using a radical sulfate ion in the presence of the picolinate anion. See supplementary information for further synthesis details of both compounds.
Figure 1. The two coordination compounds synthesized (a) copper(II) picolinate and (b) silver(II) picolinate.
Following synthesis, infrared spectroscopy was conducted on both products to confirm the successful coordination of picolinate with a central metallic atom in the oxidation state of 2+ (Table I).
Table I. Peak assignments for infrared spectra of copper(II) and silver(II) picolinate and literature values1,7. ν=in-plane vibration, β=out-of-plane vibration, γ=in-plane bending α=out-of-plane bending.
Assignment |
Ag(pic)2 wavenumber (cm-1) |
Literature value1 (cm_1) |
Cu(pic)2 wavenumber (cm-1) |
Literature value7 (cm-1) |
ν(CH)ar |
3062 |
– |
3076 |
3075 |
νas(COO–) |
1631 |
1630 |
1643 |
1646 |
ν(CC)ar |
1591 |
1600 |
1604 |
1601 |
ν(CC)ar |
1572 |
1570 |
1571 |
1570 |
ν(CC)ar |
– |
– |
1475 |
1475 |
ν(CC)ar |
– |
– |
1450 |
1448 |
νs(COO–) |
– |
– |
1350 |
1350 |
β(CH) |
– |
– |
1286 |
1286 |
β(CH) |
– |
– |
1171 |
1170 |
β(CH) |
– |
– |
1097 |
1097 |
β(CH) |
– |
– |
1049 |
1049 |
β(CH) |
– |
– |
1034 |
1032 |
βs(COO–) |
– |
– |
852 |
851 |
γ(CH) |
– |
– |
777 |
777 |
α(CCC) |
– |
– |
714 |
712 |
α(CCC) |
– |
– |
661 |
661 |
βas(COO-) |
– |
– |
– |
546 |
The electronic absorption data was collected on a spectrophotometer to characterize the electronic structure of copper(II) and silver(II) in the solid state (Figure 2).
Figure 2. The electronic absorption spectra of the solid-state copper(II) picolinate (a) and silver(II) picolinate (b) in a mineral oil mull recorded from 200 to 800 nm Spectra obtained by Brittany Amor and Leland O’Connor.
Table II. Band assignments in solid-state electronic absorption spectra for copper(II) and silver(II) picolinate.
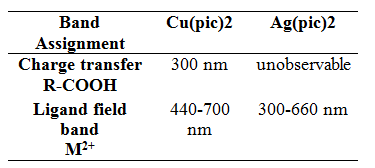
To find the molar absorptivity and observe a less-compressed spectrum, the electronic absorption of Cu(pic)2 was also obtained in the solution state (Figure 3).
Figure 3. Electronic absorption spectra of the solution state of Cu(pic)2 at a concentration of 2.5 mM, recorded with a wavelength range of 300-900 nm. Spectra obtained from Gisella Newberry and Rina Fujiwara.
Electronic spin resonance (ESR) was conducted on both products to further characterize their electronic character (Figure 4).
To confirm the electronic structure characterized with ESR and electronic absorbance, density function theorem (DFT) calculations were conducted on the copper(II) picolinate. The geometry was energetically optimized for the highest occupied molecular orbital (HOMO) and two molecular orbital diagrams were proposed: the α and β configurations (Figure 5).
Figure 5. Density function theorem calculations of the highest occupied molecular orbital in copper(II) picolinate using Spartan software.
Discussion:
While the copper(II) picolinate was synthesized in a simple ligand substitution reaction (scheme 1), the synthesis of the silver(II) picolinate involved an additional step: the oxidation of silver(I) to silver II) (Scheme 2).
Silver in the 2+ oxidation state is not commercially available because of its powerful oxidizing nature. Therefore, a strong but kinetically slow oxidizing agent, (NH4)2S2O8, oxidized silver(I) to silver(II) in the presence of the picolinate anion, an electron donor. In the absence of a Lewis base, Ag2+ in solution would immediately oxidize water and produce oxygen gas. The oxidizing power of silver(II) is due to the increased ligand splitting energy of silver compared to that of copper, following the periodic trend of increasing ligand splitting energy down a transition series5.
Although the IR spectra were not measured at wavenumbers low enough to detect the presence of copper and silver cations, both IR spectra do show that picolinate was in fact present in both products (Table I). An intense band at approximately 1630 cm-1 on both spectra indicates the asymmetric vibration of carboxylate on the aromatic ring of picolinate (Table I). These carboxylates are most likely mondentate in both picolinate compounds7. The νas(COO–) band is slightly less in the silver compound than the band observed in the copper compound (Table I). This discrepancy between the two compounds is most likely to be caused by the slightly stabilizing effect Cu2+ has on the electronic charge distribution of picolinate7. The bands present between 1600-1460 cm-1 all correlate to vibrations between two highly unsaturated carbons, in this case in an aromatic ring (Figures 8,9). The remaining peaks correlate to the in-plane and out-of-plane vibration of the aromatic ring. We cannot only confirm the success of synthesizing copper(II) and silver(II) picolinate, but that the two compounds have similar molecular structures. From the literature, we can further speculate that the structures are identical, not just similar. The IR spectrum of the copper picolinate also shows the characteristic three-band pattern of water, indicating the formation of copper(II) picolinate monohydrate.
Although the IR spectra of the two compounds appear very similar, their electronic absorbance spectra reveal differences between copper(II) and silver(II) picolinate’s electronic structure. Electronic absorbance spectral data give specific information pertaining to the ligand field and the charge transfer transitions of the analyte. In the spectra of copper(II) picolinate, two bands were observed (Figure 2a). The central atom oxidized the picolinate ligand in the formation of the coordinate covalent bond, correlating to a ligand-to-metal charge transfer. Therefore, the peak at 370 nm indicates the bond between copper and the carboxylate group of picolinate. Oxygen, not nitrogen, is oxidized by the central metal atom due to its lower ligand splitting energy, suggested by its lower position in the spectrochemical series. Located in the ultraviolet region, this charge-transfer band does not contribute to the visible color of copper(II) picolinate. However, the ligand-field band at approximately 600 nm absorbs in the orange section of the visible light spectra, giving the compound its brilliant blue color. This broad, asymmetric band is absolute evidence of copper in the 2+ oxidation state and in actuality is a composite of three broad bands. These three converged bands indicate the three electronic transitions possible when an electron is excited by a photon in a square planar coordination compound.
In contrast to the copper(II) picolinate IR spectra, there are no clear bands in the silver compound IR (Figure 2b). As a second transition series metal, silver has a higher ligand splitting energy than copper, a first transition series metal. Because of its higher ligand splitting energy, we can predict that the charge transfer band corresponding to the bond between the Ag2+ and the carboxylate group in picolinate is located at a higher wavelength for the silver compound than the copper. We were unable to observe this band due to the broad composite ligand field band. The ligand field bands are so broad in the silver compound because the differences in energies between the ground state and the photon-exited states are larger due to the increased ligand splitting energy. The maximum absorbance falls in the blue region, accounting for the orange-red color transmitted by the silver(II) picolinate synthesized. Alone, the electronic absorption spectrum of silver(II) picolinate has no obvious significance. But with a side-by-side comparison with the copper(II) picolinate spectra we can confirm the predicted increase in ligand splitting energy of silver, resulting in higher energy non-degenerate d orbitals in silver.
To observe a less compressed electronic absorbance spectrum and calculate molar absorptivity, the solution state spectra were measured for the copper(II) picolinate (Figure 3). Solution state spectra could not be obtained for silver(II) picolinate because it is almost completely insoluble and unstable when in solution.
The solution electronic absorption spectra are much less compressed than the spectra in the solid state using a mineral oil mull. The calculated molar absorptivity was approximately 50 M-1 cm-1, within the range acceptable for ligand field bands. The weak molar absorptivity, location in the visible spectra, and broad nature of the peak at 600 nm confirms it to be a ligand field band representing the presence of Cu2+. The charge transfer band was greatly compressed in the solid-state spectra in comparison the solution state: the band is unobservable at the measured wavelengths.
In both products, the electronic spin resonance (ESR) indicates two different types of electron density in an approximately 2:1 ratio (Figure 4a,b respectively). The axis parallel to the magnetic field, by convention the z-axis, showed the lower electron density, while the two perpendicular axes, x and y, were equivalent in electron density: twice as much as observed in the z-axis. The calculated electron density distribution reveals that axial symmetry is present in both compounds, consistent with a square planar geometry. From calculated μSO values (Table III), which were both close to the literature 1.73 μB value5, we can also deduce that one unpaired electron is present in the molecular orbital. This conclusion is also consistent with the d9 electron systems of copper and silver. The ESR results are in good agreement with the density function theorem calculations (DFT) of copper(II) picolinate (Figure 5). The DFT model used a fixed bond angle of 180° between the oxygens of the two picolinates complexed with the central Cu2+, which resulted in the positioning of oxygen trans relative to the nitrogen of picolinate. One unpaired electron resides in the highest occupied molecular orbital (HOMO), which therefore is also the singly occupied molecular orbital (SOMO). A one unpaired electron system further confirms a square planar geometry for the coordination compound. Picolinate, a square planar ion, enforces its geometry because of its high ligand splitting energy, therefore the geometry of the picolinate compounds is ligand imposed. The DFT calculation shows the HOMO/SOMO as the anti-bonding dx2-y2 orbital due to the destructive interference observed between the dx2-y2 interaction with π* ligand orbitals.
Following the periodic trend, the ligand splitting energy increases down the transition series from copper to gold, as already observed in solid-state electronic spectra. The composite band in Ag(pic)2 is so broad, because of the higher ligand splitting energy of Ag, that it obscures the charge transfer band in the electronic absorption spectra of silver(II), while both the ligand field and charge transfer bands are visible in the Cu2+ compound (Figure 2a). This increase in ligand splitting energy leads to the powerful oxidizing behavior of silver(II) and has an even more dramatic effect in gold(II). Although all three coinage metals have similar ionization energies and electron configurations, gold is practically unknown in the chemical world in the 2+ oxidation state. Gold has an extremely high ligand splitting energy, causing it to be a potent oxidizing agent and highly unstable. In addition, gold is later series transition metal and large in size, so relativistic effects may also contribute to the high energy of the dx2-y2 orbital. However, the contributions of relativistic effects on gold(II) are impossible to clarify because of its tremendous instability4.
Summary and Outlook:
Sara Adelman
Two coinage metal coordination compounds were synthesized using silver(II) and copper(II). Picolinate, a strong Lewis base and chelator, and therefore a strong-field ligand, served as the ligand in both compounds. These two coordination compounds were characterized and compared using a number of instrumental techniques. Infrared spectroscopy confirmed the successful coordination of a central metallic atom in the 2+ oxidation state with the picolinate ion, as well as the close similarity in the molecular geometry of the copper(II) and silver(II) picolinate. Electronic absorption spectra, in both the solid and solution phase for the copper(II) compound but only the solid-state the silver(II) compound, were compared and revealed electronic differences between the electronic structures of the two coordination compounds. In comparison to the electronic absorption of copper(II) picolinate, silver(II) picolinate has a large ligand splitting energy, explaining the powerful oxidizing behavior of Ag2+. From the electron spin resonance (ESR) of the two compounds, the electron density was determined to be equivalent in the x and y direction with a 2:1 ratio between the x and y axes to the z axis in both coordination compounds. Also, the spin only magnetic moment calculated from ESR data indicated the presence of one unpaired electron in both copper(II) and silver(II) picolinate. From the density function theorem calculations of Cu(pic)2, we can conclude that this one unpaired electron resides in the HOMO/SOMO, which is an antibonding orbital composed from the dx2-y2 orbital from the central metal atom and the π* orbital from the ligand molecule. The characterization of these two classical coordination compounds supported ligand field theorem predictions of periodic trends and observed behavior of coinage metal coordination compounds.
Agust Olafsson
In this experiment two square planar coordination complexes were synthesized with group 11 transition metal centers. Copper(II) picolinate and silver(II) picolinate formed by chelation of two picolinate ions to each metal center, due to the strong field nature of the ligand. Once synthesized the electronic and physical spectral properties were explored. IR spectroscopy, UV/Vis spectrophotometry, and ESR data showed that the two compounds were incredibly similar in structure, even though the central metal atoms were from early and late series. However, the UV/Vis did prove that the ligand splitting energy of the Mπ→Lπ* charge transfer in the silver complex was much higher compared to copper. Once the shape of the molecule was determined density functional theory with optimized bond angles showed that the molecule had one unpaired electron in the antibonding dx2-y2 orbital HOMO. Because of the one unpaired electron, it would be good to test the oxidizing ability of both compounds. If they prove to be good reducing agents, they have potential for new catalytic compounds which go through a series of 1e– transfers. The same types of experimental analyses may also be continued on other square planar chelate complexes.
Acknowledgements
The authors would like to thank Dr. Thomas J. Smith for providing thorough laboratory procedures and for obtaining the ESR spectra. The authors also wish to thank Western Michigan University for use of their ESR instrument. In addition, the authors thank Joe Widmer for his work on density functional calculations on the copper(II) picolinate compound. Also, a thank you is in order to Gigi Newbery and Rina Fujiwara for their help with synthesis and characterization of the copper(II) picolinate compound, as well as for the use of the solution and solid state UV/Vis spectra of that compound. Furthermore, the authors extend their gratitude to Leland O’Connor and Brittany Amor for allowing the authors to use their solid-state electronic absorption data.
Supplementary Information:
Figure 8. Solid-state infrared spectra of Cu(pic)2 using mineral oil mull on NaCl plates and Midac M-series FTIR spectrometer in the range 4000-600 cm-1. Obtained by Gisella Newberry and Rina Fujiwara.
Figure 9. Solid-state infrared spectra of Ag(pic)2 using mineral oil mull on NaCl plates and Midac M-series FTIR spectrometer in the range 4000-600 cm-1.
References:
(1) Fowles, G. W. A.; Matthews, R. W.; Walton, R. A. “Studies on Co-ordination Compounds of Silver (II). Part I. Magnetic and Spectral Properties of Complexes with Pyridine Carboxylic Acids. J. Chem. Soc. A. 1968, 1108-1103.
(2) Kauffman, George B. Theories of Coordination Compounds. Coordination Chemistry. 1994, 2-33.
(3) Richens, David T. Ligand Substitution Reactions at Inorganic Centers. Chemical Reviews. 2005, 105 (6), 1961-2002.
(4) Mohamed, A.A. Advances in the coordination chemistry of nitrogen ligand complexes of coinage metals. Coordination Chemistry Reviews. 2010. 254, 1918-1947.
(5) Atkins, P.; Overton, T.; Rourke, J.; Weller, M.; Armstrong, F.; Hagerman, M. Shriver and Atkins’ Inorganic Chemistry, Fifth Edition; W.H. Freeman and Company: New York, 2010; pp 473-504.
(6) Sears, Margaret. Chelation: Harnessing and Enhancing Heavy Metal Detoxification—A Review. The Scientific World Journal. 2013, 1-13.
(7) Kalinowska, M.; Borawska, M.; Swisłocka, R.; Piekut, J.; Lewandowski, W. Spectroscopic (IR, Raman, UV, 1H and 13C NMR) and microbiological studies of Fe(III), Ni(II), Cu(II), Zn(II) and Ag(I) picolinates. Journal of Molecular Structure. 2007, 419–425. 0022-2860